#
Power Generation
# Introduction
As highlighted earlier in this document, power generation is the largest source of GHG emissions in the energy economy. While considerable progress has been made in reducing GHG emissions through the implementation of cleaner electricity generation and increased energy efficiency, there will continue to be a need for on-demand, flexible options for power generation. The Power Generation TSC is focused on technologies that enable the use of AECs such as hydrogen, ammonia, synthetic fuels, and biofuels for electric power generation, and the tradeoffs between AECs and hydrocarbon-based fuels with carbon capture and storage CCS. It is anticipated that gas turbines, heat recovery steam generators (HRSGs) with duct burners, boilers, reciprocating internal combustion engines (RICE), fuel cells, and/or alternate power cycles such as supercritical CO2 operating on AECs will be some of the key technology options capable of supporting a clean electricity grid.
The use of AECs for power generation may be accomplished in two ways:
Utilization of existing power generation assets fired by traditional fuels blended AECs; and
Power generation technologies using pure AECs.
# Existing Power Generation Assets with Blended AECs
Gas turbines, fuel cells, RICE, and boilers could be ready for commercial operation with blended fuels within the next 10 years, but broad deployment will likely depend on successful, near-term field demonstrations. The degree to which the existing power generation fleet ultimately transitions to blended fuels may depend upon equipment/hardware capability, policy changes, financial incentives, and the availability of sufficient quantities of AECs at an acceptable price. Technical hurdles must be addressed to allow for safe and reliable operation of combustion assets with blended fuels. Many lab- and pilot-scale studies have previously focused on combustion characteristics and results suggest fuel blending could be accomplished with modifications to existing equipment. However, full-scale testing is needed to ensure that operation behavior is understood over the range of conditions that these machines are expected to operate. Testing at full-scale would also provide necessary information on impacts to BOP equipment, environmental control technologies (for example, selective catalytic reduction systems), operations and controls, and material capability and durability.
# Power Generation Technologies Using Pure AECs
Fuel cell operation with pure hydrogen for power generation has already passed the demonstration phase with many utility-scale installations in commercial operation in South Korea (Power Engineering 2020). However, continued improvement in fuel cell technology performance and cost are needed for fuel cell use to become more widespread in power generation applications. Additional developmental efforts to mature gas turbines, HRSGs, boilers, and RICE for operation with pure hydrogen are necessary, and with accelerated research efforts these technologies may be ready for field-demonstration before 2030. The use of ammonia with combustion or fuel cell technologies is at a more nascent stage and likely further away from field demonstration.
Major technical hurdles remain in the use for hydrogen for combustion or ammonia for fuel cells or combustion, but the development pathway for each fuel is similar and includes:
Modeling studies and design modifications;
Lab and pilot-scale studies;
Full scale testing at multiple locations for various applications; and
Definition and implementation of codes and standards.
# Key Research Questions
With increasing variable renewable power generation (wind and solar), what are the near-term and long-term market projections (demand, duty cycles) for AEC-based power generation systems?
Given the projections for electricity generation mix, what are the primary factors driving the short-term and long-term sustainability of different AEC-based power generation options (for example, heat rate, load response and flexibility, safety and environmental impacts, existing infrastructure, conversion costs, new equipment costs, geographical location)?
Which AECs and power generation technologies show the most promise for near-term needs in the ongoing energy transition and which are best for long-term sustainability of a decarbonized future?
What are the key technical challenges or knowledge gaps that must be addressed to develop and accelerate promising AEC-based power generation technologies (for example, combustion dynamics, nitrogen oxide emissions, hardware impacts)?
What are realistic development costs and timelines for these technologies to be commercially available for operation with various AECs?
Who are the key stakeholders and collaborators (for example, original equipment manufacturers, power plant engineers and operators, fuel suppliers) that must be engaged to achieve cost and performance targets that allow these solutions to be available when needed?
What are the key technical challenges associated with the safety of deploying pure and blended fuel (for example, hydrogen with natural gas or hydrogen with ammonia) in power generation equipment such as turbines and RICE, given the significant benefits arising from the use of existing assets (for example, pipelines) while lowering GHG emissions in the near term?
# Research Effort
The overall research objective for this TSC is to understand and advance power generation technologies that could support the transition to a decarbonized future by using blended or pure AECs.
A summary of the proposed research effort and associated goals is provided below.
# Research Goals
# Goal 1: Conduct market and sustainability analysis
Strategy 1: Determine key factors for understanding the potential role of AEC-based power generation technologies in a decarbonized future
- Action: Define demand and duty cycles as the projected electricity generation mix changes and additional technologies capable of providing flexibility to the power system are required. The importance of heat rate, load response and flexibility, existing infrastructure, conversion costs, new equipment costs, and geographical location will be considered.
# Goal 2: Identify and lead efforts to accelerate advanced technology development
Strategy 1: Address the need for commercial/full-scale testing
- Action: Promising AEC-based power generation technologies will be identified from the Goal 1 analysis, and key R&D needs will be determined to acceleration these technologies.
# Goal 3: Support large-scale technology and system integration
Strategy 1: Design and execute development and demonstration projects to address wholistic perspectives of AEC-based power generation
- Action: R&D work will be selected from the Goal 2 assessment and executed to address key challenges and accelerate promising AEC-based power generation technologies.
Strategy 2: Conduct technoeconomic analyses and timeline impact studies
- Action: Learning from the previous goals, costs, and timelines for commercial implementation of promising AEC-based power generation technologies will be developed.
# Goal 4: Lead efforts to assure safety of power generation equipment deploying pure and blended AEC fuels
Strategy 1. Design and execute development and demonstration projects to validate safe deployment of power generation equipment integrated with BOP components operating with pure and blended AEC fuels
- Action: Leverage existing safety codes and standards to determine safety engineering approaches and gaps that must be addressed through changes in the design, materials, and manufacturing processes.
# Anticipated Role of the LCRI in Technology Development
The TSC routinely evaluates the landscape of commercial and developing technologies relevant to the research goals. The TSC has organized technologies by anticipated role of the LCRI in technology development (see Figure 23). The TSC seeks to balance many considerations when choosing the anticipated level of activity. These considerations include projected deployment timeframe, level of effort, focus, and investment of other organizations/initiatives with similar goals, potential role in supporting various industries/sectors in decarbonization strategies, and amount of relevant information available to conduct thorough analyses, among others. Over the course of the initiative, the TSC intends to update these positions based on research results from ongoing TSC activities and energy-economy modeling results produced by the Integrated Energy System Analysis TSC.
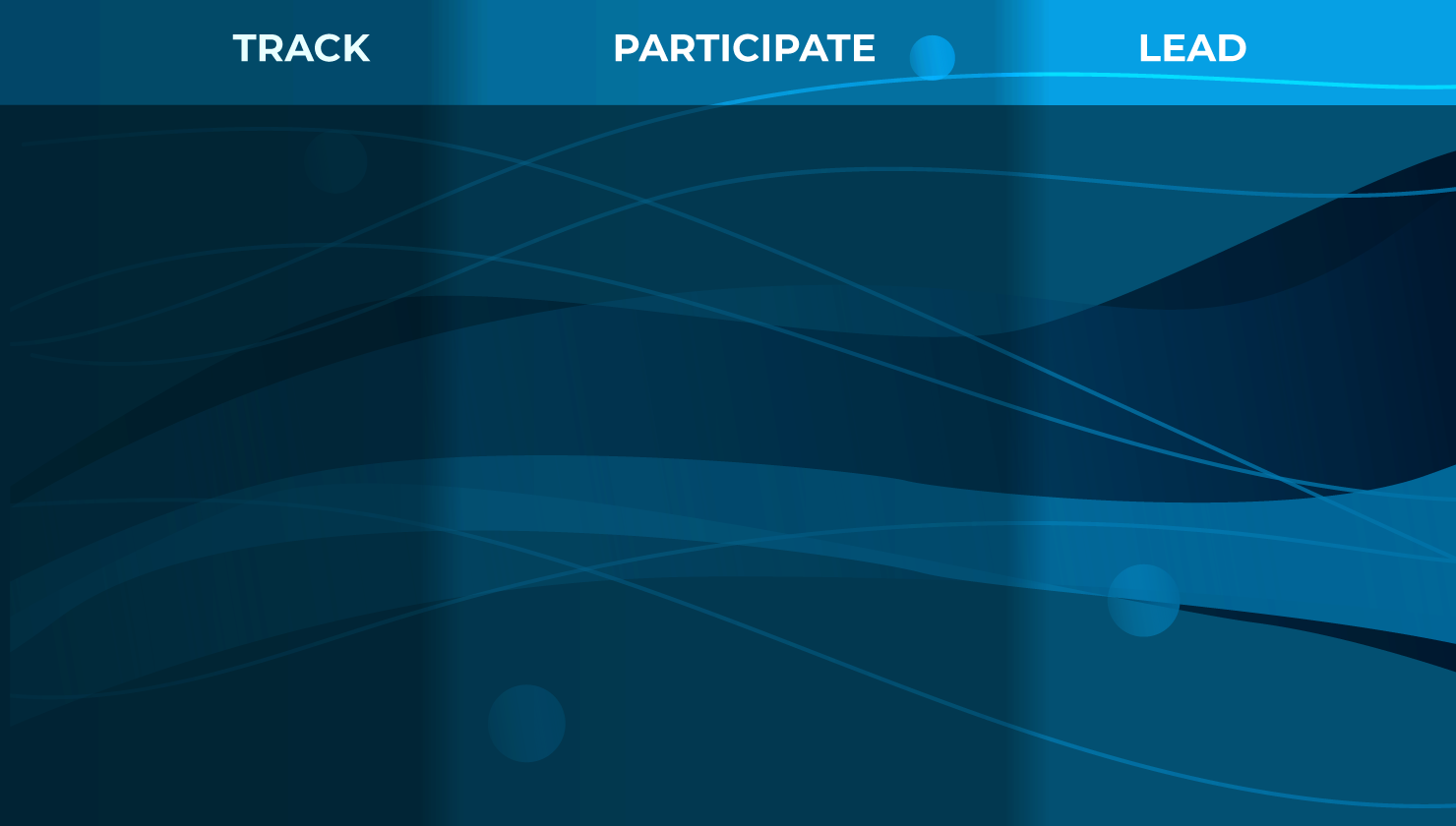
Please select "+" pushpins to see more details.
# Joint Areas of Research with Other Technical Subcommittees
The list below outlines areas where joint research with, and integration of, activities from other TSCs will be necessary.
Safety aspects and hardware (materials) impacts of handling, transporting, storing, and combusting hydrogen, ammonia, and other AECs and blended fuels.
Environmental aspects of geologic hydrogen storage.
End use drivers and the impacts of demand on low-carbon fuel delivery.
Production and supply capabilities of AECs.
System performance, techno-economic and life-cycle analysis models, and application comparisons (AECs vs. current fuels with CCS).
Cross-cutting aspects of subsystems and components used in the industrial sector and for heavy duty transportation applications that could be leveraged for utility-scale power generation.