# Perspectives on Economy-Wide Decarbonization
# Electric Sector Perspective
One potential route to produce hydrogen, and thereby other clean AEC's such as ammonia, SNG, and other synthetic fuels, is via electrolysis powered by clean electricity (often called power to gas). With a clean energy supply, decarbonization can be achieved through electrification, as previously described in Transformation 3.[1] However, for reasons which may include a need for higher temperatures, more rapid temperature ramping, system resiliency, or a need for long-duration energy storage, among others, direct conversion of processes using fuels over to the use of electricity may not be economically feasible or even possible. Therefore, the production of hydrogen from clean energy may be necessary to provide a low-carbon solution for these processes.
# Current Electricity Usage in Hydrogen Production and Indirect Electrification Opportunity
While relatively little of the 70MMt of pure hydrogen used annually across the globe (IEA 2019b) is currently produced by electrolysis, the electricity use required to produce this hydrogen is not trivial. The median electrical to hydrogen conversion efficiency, also known as the specific energy use, of an electrolyzer is currently approximately 53kWh/kg-H2[2] (Mayyas 2018). If it is assumed this is the specific energy for currently deployed electrolyzers, nearly 26.6TWh of electricity is used annually to produce approximately 0.5MMt of hydrogen today. This represents 0.1% of total electricity usage globally (IEA 2019a) or slightly more than the total electricity consumption of the state of New Mexico in 2018 (EIA 2020a).
Figure 11 shows the electricity consumption necessary for varying amounts of the current supply for pure hydrogen to be sourced from electrolysis. One interesting production level, from a carbon emission reduction standpoint, is if electrolysis were to replace hydrogen currently supplied by coal gasification. This would bring the amount of pure hydrogen supply from electrolysis to ~30% and would require 1097 TWh of electricity, representing 4.1% of 2018 global electricity demand or approximately the electricity consumption of Russia in 2017 (IEA 2021). This conversion would reduce carbon emissions by 358 MMt[3], representing a 1% reduction in worldwide carbon emissions assuming all the electricity utilized were to be sourced from electricity generation without carbon emissions. Alternatively, if 100% of current pure hydrogen demand were to come from electrolysis 3657 TWh of electricity would be needed, which would represent 88% of electricity generation in the U.S. in 2018 (EIA 2019).
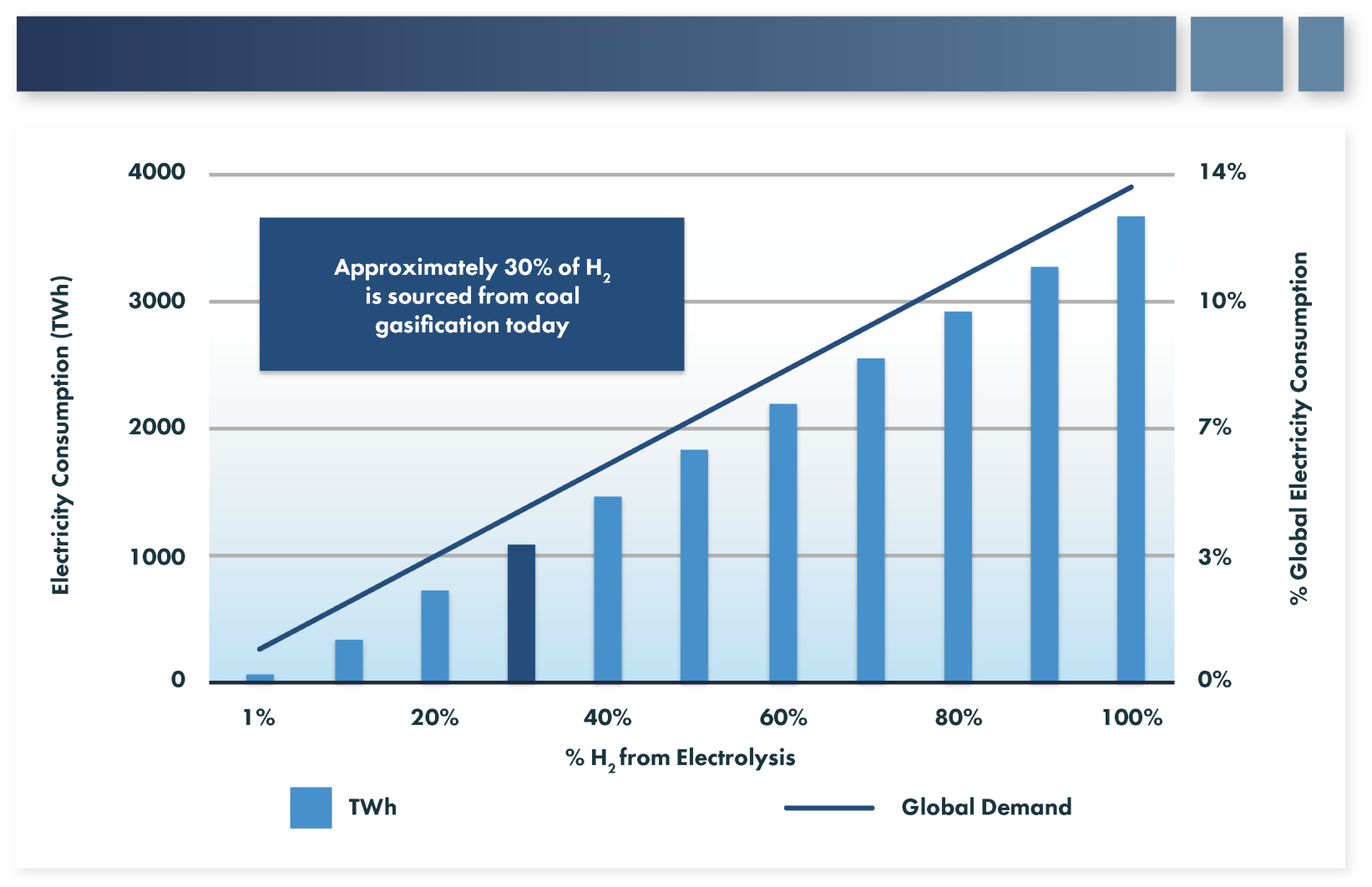
# Electrolysis Opportunity with Growth in Hydrogen Demand
There is a considerable range in the predicted growth in hydrogen demand between now and 2050, as shown in Figure 12a. The differences in demand are caused by differences in assumptions around the cost of hydrogen in the future, carbon pricing or other policies designed to reduce emissions, and the technical ability to convert certain applications over to the use of hydrogen, among other factors. However, by 2050, baseline projections for hydrogen consumption generally vary between an increase of approximately 2 times in Shell's scenario (Shell 2018) to nearly 8 times in the Hydrogen Council scenario (Hydrogen Council 2017). Figure 12b shows the projected electricity demand for pure hydrogen in 2050 for the two scenarios, assuming no improvement in electrolysis efficiency. In each case, if electrolysis supplies greater than 20% of projected pure hydrogen demand, the amount of electricity needed for pure hydrogen production exceeds 5% of the 26,672 TWh of global electricity consumption in 2018 (IEA 2019a). At the extreme, if 100% of pure hydrogen were sourced from electrolysis under the higher scenario the amount of electricity required would exceed the total electricity consumed across the world in 2018.
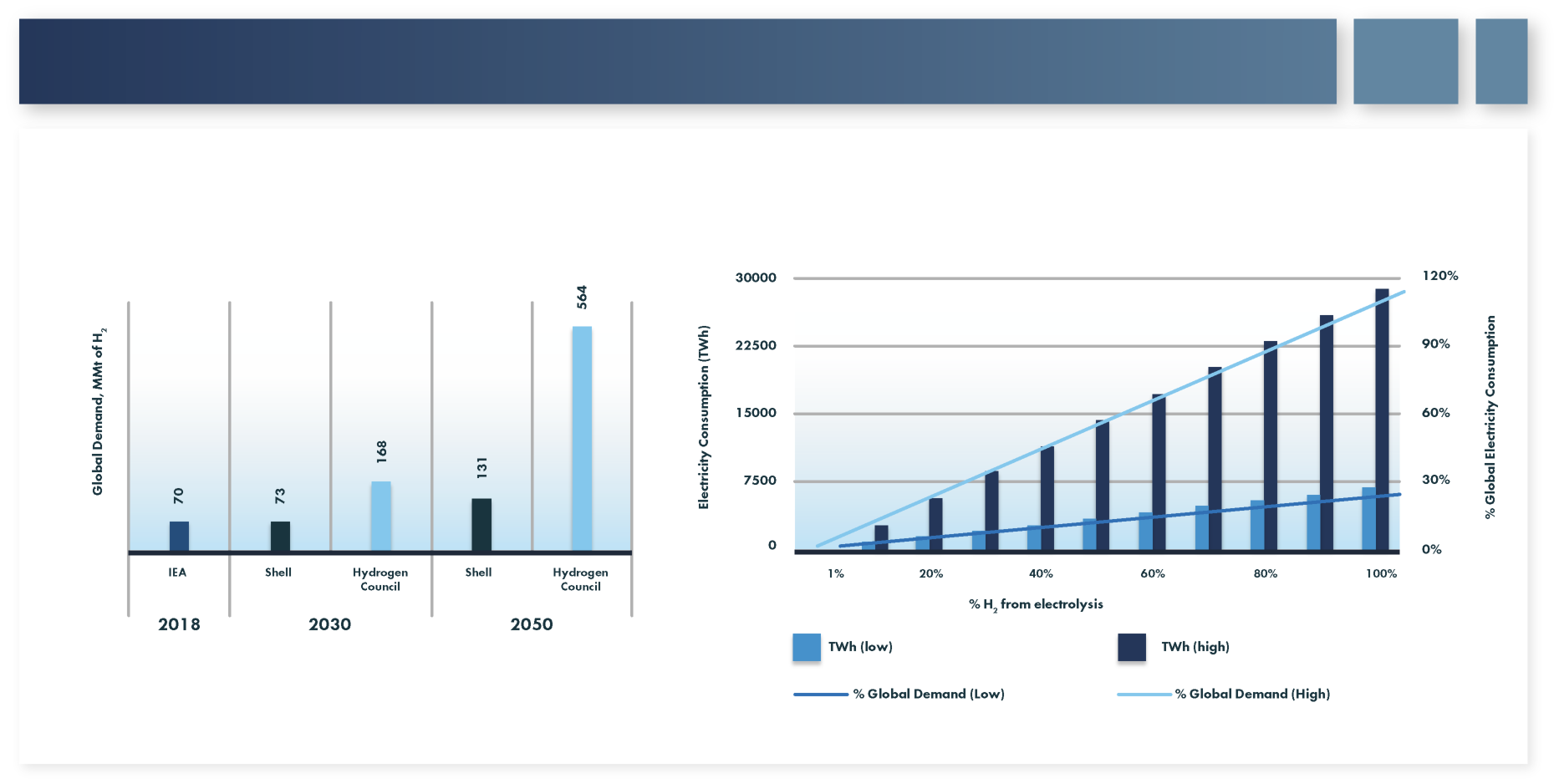
# Natural Gas Sector Perspective
Achieving NZE by mid-century will require deep carbon reductions across the entire economy and all forms of energy, but that does not mean that there is not a role for fossil fuels or gas infrastructure in such a future. Today, natural gas supplies a reliable and affordable source of energy to all major sectors of the economy. However, to achieve mid-century GHG emission reduction goals most deep decarbonization studies and pathway analyses conclude that economy-wide fossil fuel consumption must decline—but not disappear altogether. This poses challenges and opportunities for natural gas utilities (also referred to as local distribution companies, or LDCs), whose regulated business models currently center around procuring reliable and affordable supplies of natural gas and delivering them safely over gas networks to end-users.
In the near term, LDCs will continue to make progress on reducing CH4 leakage throughout their systems (as shown previously in Figure 4) while helping customers become more energy efficient through building retrofits, implementation of residential technologies with improved efficiency, and deployment of new technologies such as advanced thermal heat pumps and hybrid heating systems to reduce gas demand across the economy. Additionally, many LDCs are actively pursuing programs to blend renewable gas into their pipelines to reduce carbon intensity. Through steps already taken, natural gas consumption in the U.S. has already been reduced by nearly 50% across all sectors of the economy, on a per capita basis, relative to levels in the early 1970's (Figure 13).
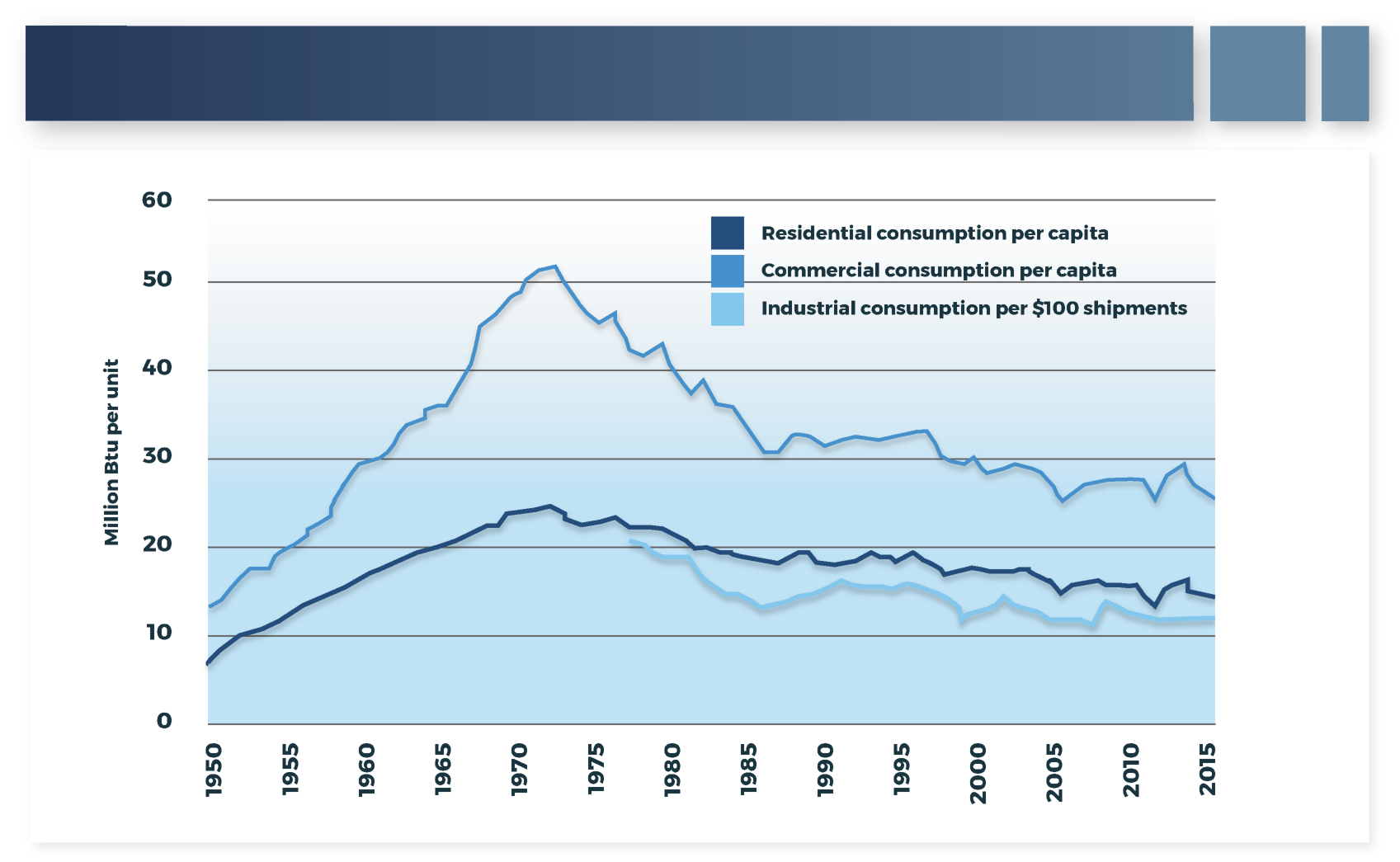
As the electricity sector evaluates transitioning from fossil fuels to emissions free resources and as other natural gas end uses in the transportation and industrial sectors increasingly electrify, the existing natural gas supply and infrastructure will play a key role in this energy transition. Natural gas power plants will play a critical role in maintaining the reliability and resiliency of electric supply as increasing amounts of variable renewables are integrated onto the grid. Further, natural gas plants equipped with CCS will continue to be an option for reliable and resilient electricity supply in a fully decarbonized electricity sector. Additionally, blending of RNG, SNG, and/or hydrogen into the existing gas network will help to begin to decarbonize applications which currently use natural gas while serving as a long duration storage asset for excess power produced by variable renewable resources. It is estimated that by 2040 there is a technical renewable gas potential of 4 quadrillion Btu (Quads)/year and a total annual resource of 14 Quads that could be realized in the U.S., which is nearly equal to the annual amount of current residential natural gas demand (AGF 2019). These sources could be further complemented by low-carbon gaseous energy in the form of hydrogen or synthetic methane produced by power-to-gas facilities.
The natural gas system has several characteristics that provide inherent resiliency, including the natural stability of most fuels that enable long-duration energy storage capabilities and operational flexibility, as well as underground assets that are less likely to be disrupted by extreme weather events or other natural disasters. Leveraging the existing natural gas infrastructure, adapting it for low-carbon resources, and more tightly integrating it with the electric system may be a path to maintaining energy system reliability and resiliency more affordably than a single energy carrier system. This is particularly important given the increased reliability risks such as extreme weather (wildfires, hurricanes, polar vortex) and potential cyberattacks and increases the optionality for energy delivery to end uses.
For more on electrification, please see EPRI's U.S. National Electrification Assessment, product ID 3002013582 (opens new window). ↩︎
This median value is approximately the median value for both alkaline and proton exchange membrane (PEM) electrolyzers. For greater detail on the differing types of electrolyzers, please see the EPRI report Program on Technology Innovation: Prospects for Large-Scale Production of Hydrogen by Water Electrolysis, product ID 3002014766 (opens new window). ↩︎
This figure is based upon carbon emissions of 19 MMtCO2/MMtH2 for coal gasification (IEA 2019b). ↩︎