#
Delivery and Storage
# Introduction
Today's energy system relies on a complex network of infrastructure to deliver energy and meet dynamic demand. As multiple sectors of the energy economy transition towards a decarbonized future, a more tightly integrated energy system will become even more critical in leveraging electricity, gases, liquids, and existing energy infrastructure. Wide-spread adoption of AECs and fuels will impact transportation and delivery of energy which must be accomplished in an affordable, reliable, and resilient manner. Existing and proven infrastructure will play a key role in enabling AECs but must be evaluated to ensure adequacy and durability of service. In addition, development of new, purpose-built infrastructure will ensure the safety and reliability of energy delivery across the economy. The objective of the Delivery and Storage TSC is to determine the potential impact that AECs may have on the operations, codes, standards, and materials used for existing infrastructure, and whether that infrastructure can be safely retrofitted and repurposed or if new built-for-purpose infrastructure is required to safely meet future demand for AECs.
The deployment of low-cost, reliable infrastructure technologies—including validation of existing or new materials and components—and solutions could enable multiple pathways for reducing CO2 emissions for different sectors of the economy. These pathways range from capturing CO2 from industrial sources and storing it deep underground to integrating AECs and fuels such as hydrogen, ammonia, biofuels, and/or synthetic fuels. Each pathway could potentially require a different storage and delivery system to transport these AECs, as well as captured CO2 from the point of production, to the end user or sequestration site.
The vision of what this storage and delivery system looks like and its optimal configuration depends on the future energy mix; however, leveraging the existing energy infrastructure to enable the use of AECs provides a tremendous opportunity that could prove economical and reduce barriers to broad-scale adoption. The repurposing of existing natural gas pipelines is already being explored by European transmission and distribution system operators with initial efforts of blending hydrogen into pipelines (Gas for Climate 2020). Existing transmission and delivery networks are comprised of varying types of new and vintage materials which will be impacted differently by AECs such as hydrogen. Therefore, technical questions remain with regards to impacts of these AECs on pipelines and geologic storage facilities. The feasibility and cost of retrofitting existing gas and liquids infrastructure will need to be assessed.
In some cases, retrofitting infrastructure may not be the most cost-effective mechanism for deploying AECs, either due to limitations of blending or conversion or from the ability to meaningfully decarbonize. As shown in Figure 21, blending hydrogen with natural gas does not result in an equivalent reduction in CO2 emissions from end use applications. Many research projects are underway worldwide to address the opportunities and challenges with blending hydrogen into existing natural gas infrastructure. Despite the limited CO2 emissions reduction associated with lower hydrogen blends, existing infrastructure could support broader deployment of hydrogen in the near term by increasing demand of the fuel compared to its use today (which is primarily limited to petroleum processing and fertilizer production). This TSC will assess the tradeoffs between leveraging existing infrastructure versus building new infrastructure.
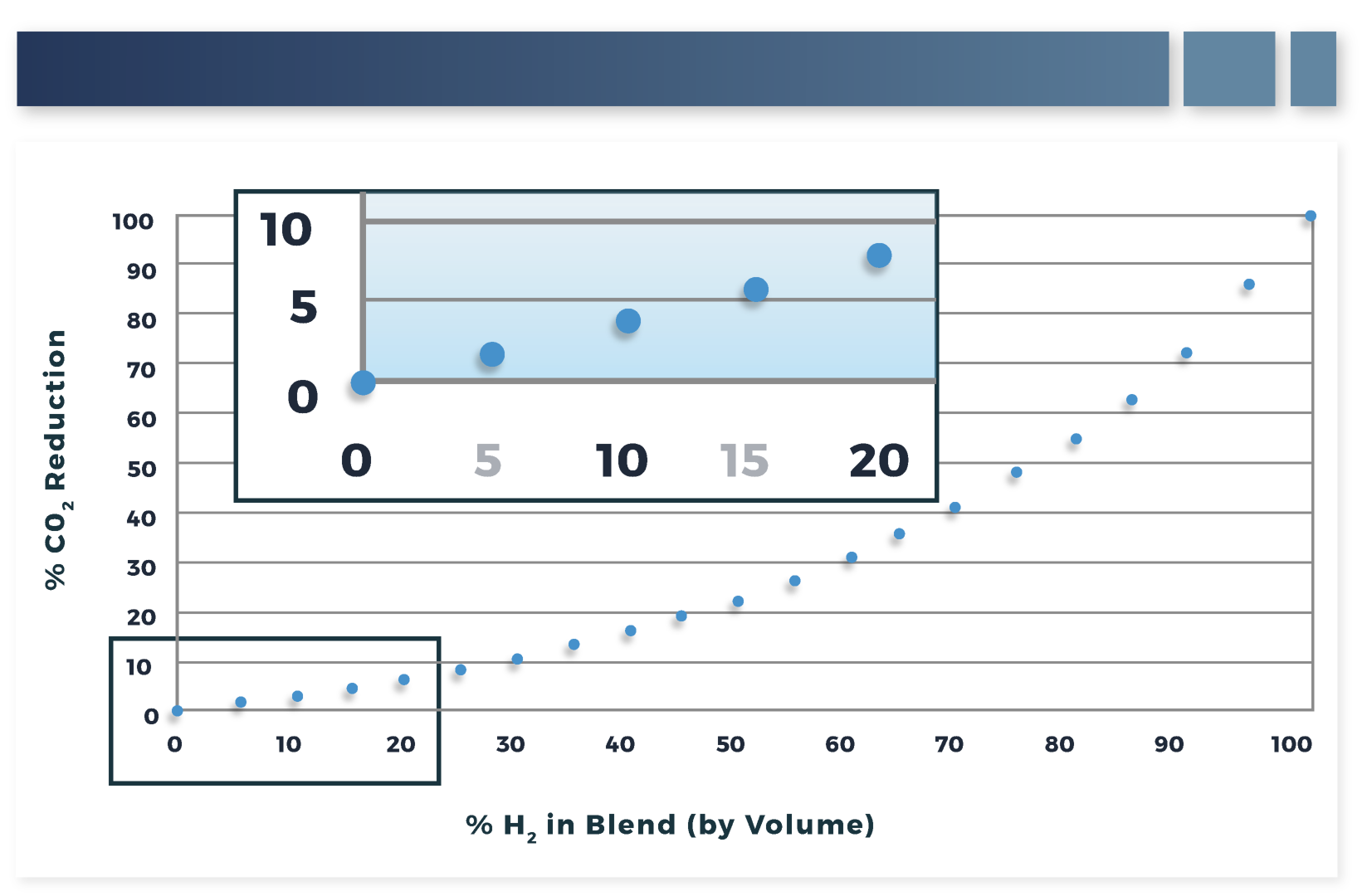
Additional options for delivery and storage of AECs includes building out new infrastructure such as hydrogen-specific pipelines (which already exist in the U.S.) as well as the use of emerging technologies such as materials-based storage or liquids-based storage. Material-based storage solutions such as metal hydrides, adsorbents, carbon nanostructures, or liquid organic hydrocarbons show promise for hydrogen storage because they allow for denser, more compact storage, and storage at near ambient temperatures and at lower pressure.
Biofuels, including RNG and drop-in synthetic fuels, benefit from the ability to leverage existing gas and liquids infrastructure due to direct compatibility with fossil fuel-based hydrocarbons. Storage and delivery of these fuels will not be an area of focus for this TSC as technologies and solutions are available and mature.
# Key Research Questions
Which AECs and fuels will be readily accepted and embraced by consumers?
Will the downstream end users demand the fuel?
Can the upstream producers supply enough fuel?
What are cost-effective ways to deliver and store these fuels and capture and sequester CO2?
What role could existing delivery and storage networks serve in increasing the adoption of AECs as the energy system transitions to a lower carbon future?
How might delivery and storage networks adapt or evolve to meet 2050 consumer demand and expectations? What transitions will need to occur in the next 30 years to enable this future?
To what extent can the existing hydrocarbon storage and delivery system infrastructure be retrofitted to cost-effectively accommodate an AEC while also delivering value towards deep carbon reductions? What are the tradeoffs that might drive towards the deployment of a completely new system?
What new delivery and storage infrastructure will be needed in the future to support the transition to new fuels?
What emerging storage technologies are being developed for stationary applications as well as large-scale storage?
# Research Effort
The Delivery and Storage TSC intends to organize research according to four primary goals as outlined below.
# Research Goals
# Goal 1: Identify critical delivery and storage requirements of promising decarbonization pathways
Strategy 1: Develop a global delivery and storage technology evaluation process
- Action: Review global approaches for delivery and storage of AECs and CO2 (captured at point sources or using DAC). Review strategies for delivering and storing energy, hydrogen-natural gas blends, ammonia, CO2, and liquid organic carriers. Additional fuel pathways may be considered based on input from the other TSCs.
Strategy 2: Conduct scenario analyses and planning evaluations
- Action: Using input from other TSCs, map decarbonization pathways to key end use sectors. Define critical operating parameters including total and peak energy demand, demand response, and production/delivery rates and pressures for scenarios. Develop scenarios for specific geographic regions, as appropriate.
# Goal 2: Conduct impact assessments
Strategy 1: Identify impacts and gaps
Action: Conduct reviews and state-of-the-art analyses for each fuel pathway identified and prioritized. Key technical areas that will be investigated may include:
Integrity of legacy infrastructure
Retrofit challenges
Blending, batching, and separation
Fuel monitoring
Fuel metering
Health, safety, and environmental aspects
Codes and standards
Policies, regulations, and incentives
Geo-storage simulation tools for hydrogen storage
Above and below ground storage tanks
Strategy 2: Establish testing and validation projects
- Action: Once gaps in knowledge are identified, additional modeling, lab- and pilot-scale projects may be proposed to characterize and assess impacts further. Testing and validation projects will be informed using the parameters from Listing 1 (not all parameters may be applicable to each low-carbon fuel).
Impact on energy content/physical properties
- Caloric value
- Wobbe Index (gases)
- EOS for fuel mixtures/blends
EH&S impacts
- Explosivity, flammability, Ignitability
- Fuel odorization (gas)/colorization (liquids)
- Leak detection
- Emergency response
Operational impacts
- Transmission and delivery system Integrity
- Changes in operating conditions
- Pipe, valve, and seal performance
- Meter accuracy and performance
- Leak detection
- Blending and batching
Impact on underground storage facilities
- Well and wellhead integrity
- Tubing, packers, seals, and cements
- Geologic containment and inventory losses
- Hydrogeochemical interactions
- Biological conversion and fuel contamination
Impacts on regulation and standards
- Applicability of existing regulations
- Applicability of existing standards
Impact on materials of construction
- Embrittlement
- Corrosion
- Erosion
- Permeation
- Failure analysis
Impacts on compressors and pump stations
- Performance
- Design
- Size
- Ancillary power
Impact on above ground storage assets
- Integrity of bulk storage tanks (fuel terminals)
- Ancillary pipes, valves, pumps, and seals
- Integrity of filling station tanks (distribution)
- Ancillary pipes, valves, pumps, and seals
- Inventory losses/control (cryogenic – boil off)
- Leak detection
Maintenance impacts
- Repairs and rehabilitation
- Frequency of maintenance/inspection
Customer billing
- Billing and credits for caloric value
Impact of fuel blending/contamination
- Separation technologies
- Centralized versus decentralized blending
# Goal 3: Understand drivers, opportunities, and challenges of future infrastructure requirements
Strategy 1: Conduct technoeconomic assessments of retrofitting existing infrastructure
- Action: Identify and evaluate technologies needed for all delivery and storage components including, but not limited to, transmission and distribution systems, underground storage facilities, above ground tanks, compressors, pumps, and conditioning/separation equipment. Resulting changes in operations, maintenance, codes and standards, and environmental, health, and safety will also be identified and evaluated to enable the use of each AEC.
Strategy 2: Conduct technoeconomic assessments of new infrastructure
- Action: Identify and evaluate technologies needed to construct new delivery and storage infrastructure including, but not limited to, transmission and distribution systems, underground storage facilities, above ground tanks, compressors, pumps, and conditioning/separation equipment. The need for new operations, maintenance, codes and standards, and environmental, health, and safety will also be identified and evaluated to enable the use of each AEC.
# Goal 4: Identify and lead efforts to accelerate advanced technology development
Strategy 1: Identify and advance emerging delivery and storage technologies
Action: Monitor emerging technologies to identify innovative materials and processes
Action: Design bench or small-scale demos to evaluate feasibility and accelerate adoption of new technologies as needed
# Anticipated Role of the LCRI in Technology Development
The TSC routinely evaluates the landscape of commercial and developing technologies relevant to the research goals. The TSC has organized technologies by anticipated role of the LCRI in technology development (see Figure 22). The TSC seeks to balance many considerations when choosing the anticipated level of activity. These considerations include projected deployment timeframe, level of effort, focus, and investment of other organizations/initiatives with similar goals, potential role in supporting various industries/sectors in decarbonization strategies, and amount of relevant information available to conduct thorough analyses, among others. Over the course of the initiative, the TSC intends to update these positions based on research results from ongoing TSC activities and energy-economy modeling results produced by the Integrated Energy System Analysis TSC.
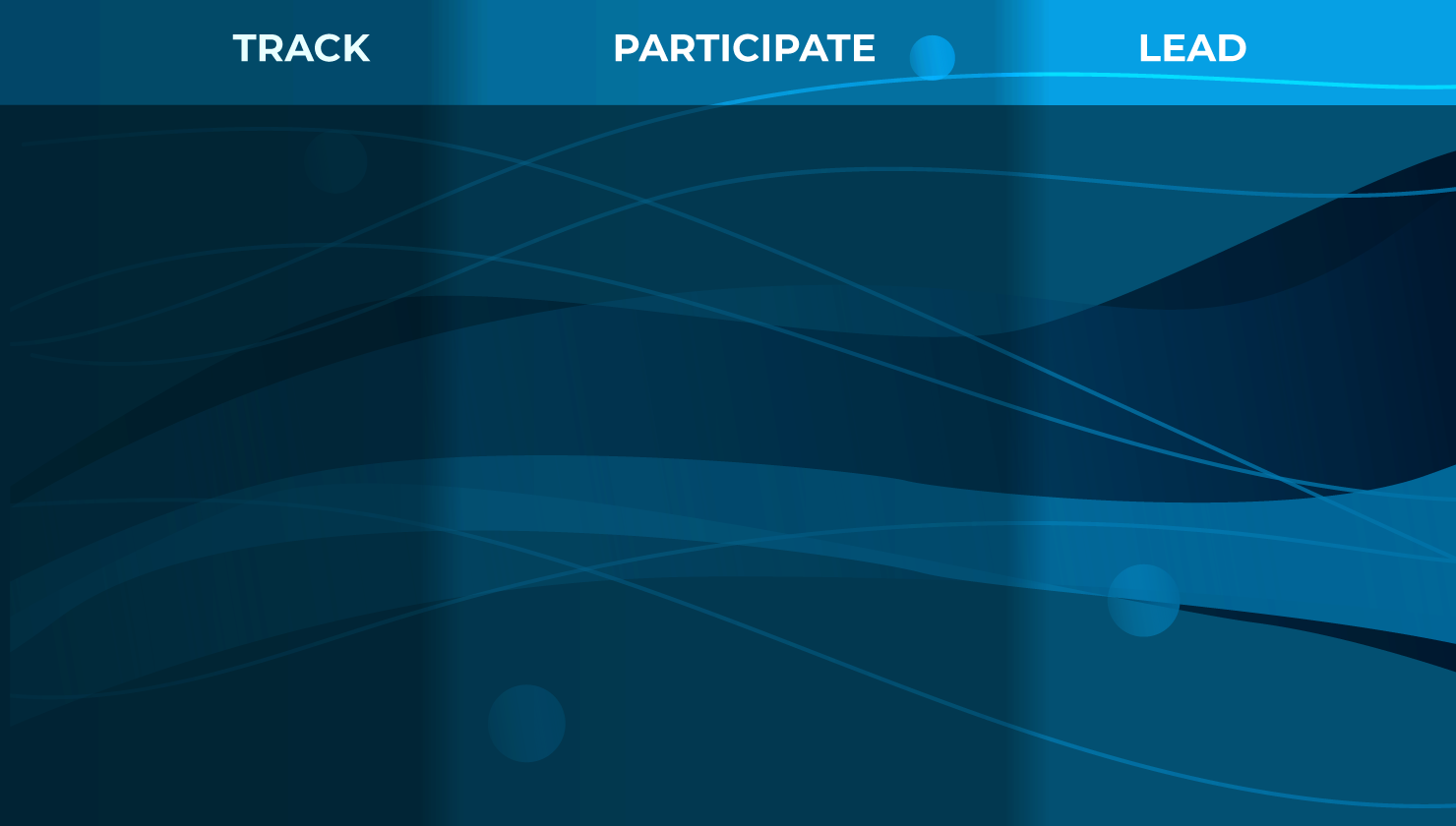
Please select "+" pushpins to see more details.
# Joint Areas of Research with Other Technical Subcommittees
The list below are areas where joint research with, and integration of, activities are anticipated from other TSC's.
Safety aspects of handling, transporting, and storing hydrogen, ammonia, and other AECs.
Environmental impacts of AEC and CO2 storage.
AEC end use drivers and impacts of demand on AEC delivery.
Production/supply capabilities and impacts of AEC and fuel availability.